Article Access Statistics | | Viewed | 39920 | | Printed | 792 | | Emailed | 14 | | PDF Downloaded | 790 | | Comments | [Add] | | Cited by others | 9 | |
|
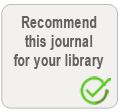
|
|
|
Year : 2004
| Volume
: 6 | Issue : 23 | Page
: 73-85 |
|
Effects of low frequency noise up to 100 Hz |
|
M Schust
Federal Institute for Occupational Safety and Health, Berlin, Germany
Click here for correspondence address
and email
|
|
 |
|
This
review concentrates on the effects of low frequency noise (LFN) up to
100 Hz on selected physiological parameters, subjective complaints and
performance. The results of laboratory experiments and field studies are
discussed in relation to the thresholds of hearing, of vibrotactile
sensation and of aural pain. The effects of LFN may be mediated trough
different ways. Temporary or permanent hearing threshold shifts seem to
be due to acoustic stimuli above the individual hearing threshold.
However, non-aural physiological and psychological effects may be caused
by levels of low frequency noise below the individual hearing
threshold. The dynamic range between the thresholds of hearing and of
aural pain diminishes with decreasing frequency. This should be taken
into account by the setting of limits concerning the health risks.
Sufficient safety margins are recommended. The use of a frequency
weighting with an attenuation of the low frequencies (e.g. G-weighting)
does not seem to be appropriate for the evaluation of the health risks
caused by LFN up to 100 Hz. It may be proposed to measure third octave
band spectra or narrow band spectra. A comparison with the known human
responses caused by the measured levels and frequencies could help to
evaluate the health risks. Some proposals for further investigations
were given:
(1) experimental methods to discover the ways mediating the effects of
low frequency noise,
(2) consideration of the individual hearing threshold or hearing
threshold shift and of the vibrotactile threshold in the low frequency
range to be able to judge the effects,
(3) consideration of combined body vibration caused by airborne low
frequency noise or by other sources,
(4) modelling to analyse the transmission of the acoustic energy from
the input into the body to the structures containing sensors,
(5) consideration of probable risk groups like children or pregnant
women. Keywords: infrasound, hearing threshold, vibrotactile perception, aural effect, non-aural effect, complaint, performance
How to cite this article: Schust M. Effects of low frequency noise up to 100 Hz. Noise Health 2004;6:73-85 |
Introduction | |  |
Although
some comprehensive reviews have been published in the past decades
(Westin 1975, Harris et al. 1976, Tempest 1976, Broner 1978, Johnson
1982, Landstroem et al. 1993, Berglund et al. 1996), this article is
supposed to supplement the overview with some contemporary publications.
It also includes older publications which were not mentioned in other
review articles or were not described there in detail. The presented
review concentrates on the effects of low frequency noise up to 100 Hz
on selected physiological parameters, subjective complaints and
performance. The influence on the loudness judgement and the annoyance
is not taken into account. Animal experiments do not receive attention,
too. Some graphics ease the interpretation of the scientific results.
Sensation of Low Frequency Noise (LFN) - the thresholds of hearing, vibrotactile perception and aural pain | |  |
The knowledge of the hearing threshold is essential for the analysis of effects of LFN. [Figure - 1]
shows the thresholds up to 250 Hz measured by different authors
(Robinson et al. 1956, Corso 1958, Yeowart et al. 1967 and 1974, Whittle
et al. 1972, Landstroem et al. 1983, Verzini et al. 1999). The
inclusion of further data would probably not change the trend obvious
from [Figure - 1].
There is only little data with a fairly variable range based on studies
of about 260 persons aged between 16 years (Robinson et al. 1956) and
70 years (Whittle et al. 1972). All published results were given as mean
values and standard deviations. No but one article presented the median
values and/or percentiles and/or extreme values. Robinson et al. (1956)
supplied the median values, which were strongly related to the mean
values, perhaps because of the large study group of 120 subjects. In
order to generate a range of representative thresholds in [Figure - 1],
standard deviations reported were added to the highest mean value and
subtracted from the lowest mean value. Perhaps, the thresholds varied
because of the used measuring method and the between- and within-subject
differences. The maximum ranges given in [Figure - 1]
varied between 20.1 dB and 29 dB at 4, 5, 25, 32, 40, 50, 75 and 100
Hz. The variability of the individual thresholds of the study
participants was probably larger, but, as mentioned above, the authors
did not report on the extreme values. Landstroem et al. (1983)
investigated the threshold of "vibrotactile" perception. The results
suggest no differences between deaf and hearing subjects. Therefore, the
mean values of both groups were presented in [Figure - 1].
The subjects described a frequency-dependent sensation of vibration of
different parts of the body (lumbar, buttock, thigh, calf).
Only one paper was found with information about the threshold of aural pain (von Gierke et al. 1976, see [Figure - 1]).
Aural effects of low frequency noise | |  |
Although
there are different opinions concerning the role of the temporary
threshold shift (TTS) as a predictor of the permanent threshold shift
(PTS), it is assumed that a better method does not exist at present. The
TTS is not appropriate for calculating the individual noise induced
hearing loss, but it is useful for predicting the PTS of groups of
persons exposed to noise of certain levels and types (Sataloff et al.
1993). [Figure - 2]
summarises the scientific results regarding the aural effects like TTS,
PTS and a sensation of pressure in the ear in relation to the hearing
threshold and the threshold of aural pain.
A number of authors obtained temporary threshold shifts in
laboratory experiments or field studies. Alford et al. (1966) and
Jerger et al. (1966) found TTS (10 dB - 22 dB) in 11 of 19 subjects
after 3 minutes repeated exposure to 119 dB - 144 dB / 2 Hz - 12 Hz. The
TTS was observed in the hearing frequency range from 3 kHz to 8 kHz.
Nixon (1973) reported on TTS (20 dB - 25 dB) in one of three
participants caused by exposure to 135 dB / 18 Hz (6 times 5minutes
exposures) and 140 dB / 14 Hz (steady exposure, duration 5 min - 30
min). Johnson (1973, cited in Johnson 1982) recorded TTS (8 dB) in the
hearing frequency range from 2 kHz to 6 kHz due to exposure to 140 dB / 4
Hz, 7 Hz, 12 Hz in one of eight subjects (duration 5 min). A prolonged
exposure time (30 min) caused TTS from 14 dB to 17 dB (one exposed
subject only).
Mills et al. (1983) obtained TTS of different
degrees and depending on the frequency of the noise (octave band noise,
centred at 63 Hz, 125 Hz or 250 Hz) in 52 subjects. A 24-hourexposure
to 84 dB(A) led to TTS from 7 dB to 15 dB in the frequency range from
300 Hz to 500 Hz. An 8-hour-exposure to 90 dB(A) caused TTS from 12 dB
to 17 dB in the frequency range from 250 Hz to 700 Hz. Tonndorf (1950)
reported on temporary hearing impairments determined by tuning-fork test
in employees which worked in engine rooms of submarines (infrasound 10
Hz - 20 Hz), but no sound pressure level was given.
In contrast, no TTS
was found by the following authors: Slarve et al. (1975) recorded no
TTS in four subjects after exposure to pure tones for a period of 8
minutes. The frequencies ranged from 1 Hz to 30 Hz (125 dB - 144 dB).
Johnson (1973 and 1980) found no TTS after various exposure conditions
(126 dB - 171 dB / 0.6 Hz - 10 Hz / 1 min - 26 min / 1 - 16 subjects).
Mohr et al. (1965) applied several different exposure conditions (see
paragraph "subjective complaints"). The authors discovered no effects on
the hearing threshold even due to the exposure to the highest levels
(narrow band noise, overall sound pressure levels 149 dB - 154 dB /
maxima at 2 Hz - 10 Hz for 2 minutes, tests 9, 10 and 11). However, it
is difficult to interpret the results, because it is not clear, which
subject wore ear protectors for which period of the exposure.
Several investigations revealed subjective aural complaints.
Karpova et al. (1970) reported on pressure in the ear after exposure to
industrial infrasound (5, 10 Hz / 100, 135 dB) for 15 minutes. Slarve
et al. (1975) described similar effects. Subjects told painless pressure
in the ear during 8 minute exposure to 144 dB / 1 Hz - 20 Hz. A
"sensation reflecting pressure build-up in the middle ear" occurred in
the tests number 9, 10 and 11 of Mohr´s experiments (see above) during
exposures without ear protection, whereas three of the five persons also
described a "tympanic membran tickle sensation". Two of three subjects
experienced middle ear pain during "brief" periods without ear
protection exposed to narrow band noise, overall sound pressure levels
143 dB - 145 dB / maxima at 25 Hz - 40 Hz (tests 12, 13, 14).
Only one epidemiological study of permanent hearing impairments
could be found. Doroshenko et al. (1983) investigated 216 compressor
operators exposed to infrasound (91 dB - 119 dB) and combined steady
noise within the hearing frequency range (84 dB(A) - 97 dB(A)) for a
daily period of 6.5 hours in a cross sectional study. The control group
consisted of 220 workers exposed to industrial noise (93 dB(A) - 106
dB(A)) without any infrasound. The duration of exposure lasted from 1
year to 20 years. The mean age ranged from 20 to 50 years. Combined low
frequency and steady noise exposure caused significantly increased
hearing thresholds verified by tonal audiometry as well as deteriorated
intelligibility of whispered speech in comparison with the isolated
industrial noise exposure. The differences enlarged with the length of
exposure.
Non-aural effects of low frequency noise | |  |
LFN can cause a lot of non-specific physiological reactions, subjective complaints and an impairment of the performance. The [Figure - 3][Figure - 4] show the results of numerous studies in relation to the hearing threshold and to the threshold of aural pain.
Vascular, respiratory and endocrine effects, balance and visual disturbance
Danielsson
et al. (1985) investigated the effect of LFN on blood pressure, heart
frequency and serum cortisol. 20 male study participants were exposed to
pure tones at different levels (95 dB, 110 dB and 125 dB) and
frequencies (6 Hz, 12 Hz and 16 Hz) for 20 minutes in the first series
of experiments. In the second set of experiments, a one-hour exposure
(125 dB, 16 Hz) was followed by a silent control period. On alternate
days, the same subjects were exposed to either infrasound (125 dB / 16
Hz) or a so called control exposure (50 dB / 50 Hz, just 5 dB above the
mean hearing level). The one-hour exposure to LFN led to a significantly
increased diastolic blood pressure and a significantly decreased
systolic blood pressure in comparison with the control exposure. No
significant changes of the heart rate and serum cortisol were obtained.
Landstroem et al. (1983) exposed 10 normal hearing and 10 deaf subjects
to 115 dB / 6 Hz for 20 minutes. In normal hearing volunteers changes of
EEG patterns - interpreted as diminished wakefulness -, alterations of
systolic and diastolic blood pressure and of heart rate were observed.
These effects were not found in deaf persons. No differences in
vibrotactile sensation were detected between both groups. Therefore the
authors attributed the observed physiological effects to cochlear
stimulation. Karpova et al. (1970) obtained the following physiological
reactions caused by 15 minutes exposure to 5 Hz and 10 Hz simulated
industrial infrasound (100 dB and 135 dB): significantly decreased
respiration rate, "depression of the encephalic haemodynamics", changes
of EEG patterns, increased heart rate, reduced heart muscle contraction
strength. Wysocki et al. (1980) reported on tendencies of decreased
heart rate, diminished electrical conductance which may result from the
peripheral vascular changes and reduced skin temperature. 40 subjects
were exposed to a low frequency spectrum typical for vehicles (control
group: 20 subjects, no exposure). Evans et al. (1972) recorded vertical
nystagmus and described a subjectively reported "feeling of body sway"
in 25 subjects exposed to pure tones (2 Hz - 10 Hz) above 130 dB. The
effects were primarily pronounced at 7 Hz. The authors developed a
threshold curve for vertical nystagmus induced by a 7 Hz binaural
signal. No level of the infrasound (1 Hz - 20 Hz / 115 - 120 dB) caused
any visual disturbance. Takigawa et al. (1988) examined the influence of
infrasound (5 Hz and 16 Hz, 95 dB, 5 minutes) on the control of upright
standing posture. The authors concluded that the excitability of the
vestibulum seemed to be accelerated by LFN, whether or not the subjects
perceived any sensations. Doroshenko et al. (1983, methods see above)
reported on significantly abnormal findings regarding the vestibular
functions in the exposed group (test of statokinetic function, calorific
test, rotational test). Waye et al. (2002) exposed 32 subjects to a low
frequency noise and a reference noise with a flat frequency spectrum at
the same A-weighted sound pressure level (40 dB(A)). For the LFN, sound
pressure levels in the frequency region of 31.5 Hz to 125 Hz were
added. Higher cortisol levels (six saliva samples during the two-hour
exposure) were associated with high sensitivity to noise and being
exposed to LFN (significant interaction). This association was not found
for the reference noise.
Subjective complaints
Slarve
et al. (1975) exposed 4 subjects to a low frequency spectrum which
contained pure tones (1 Hz - 30 Hz, 125 dB - 144 dB) for 8 minutes. The
study participants reported on voice modulation and body vibration
(abdominal, chest). Harris et al. (1978) exposed 40 subjects to 7 Hz /
125 dB, 132 dB and 142 dB, partly combined with 110 dB low frequency
background noise. The authors mentioned spontaneous complaints in six
study participants following exposure to 7 Hz / 142 dB
(vibrationsensation, pressure in the ear, inability to concentrate).
Verzini et al. (1999) reported on a feeling of vibration, pressure and
annoyance in the head, the ears and the nape during exposure to 10 Hz /
110 dB tones or to a similar LFspectrum. The results of Slarve et al.
(1975), Harris et al. (1978) and Verzini et al. (1999) correspond with
the findings of Landstroem et al. (1983) that the sensations of
vibrations caused by airborne noise occur about 20 dB above the hearing
threshold (see [Figure - 1]).
The investigations of Ising et al. (1979 and 1980) were the only
experimental ones which used prolonged exposures up to 8 hours for 10
days (3 Hz - 24 Hz / 110 dB). The subjects reported on a lack of
concentration, annoyance, tiredness, tense, irritability and
restlessness. Waye et al. (2002, see above) did not find a significant
difference between LFN and the reference noise for the mood dimensions
or for the subjective symptoms rated by questionnaires. However, the
anxiety of the subjects estimated with the Trait and State Spielberger´s
scales modulated the subjective judgements (semantic differential
scales). For example, there was a strong relationship between the trait
"anxiety" and the acceptability to the tone exposure. Landstroem et al.
(1988) studied the effects of different levels of LFN in two types of
lorries in a field investigation with 13 lorry drivers. Subjectively
rated increased fatigue was more pronounced when driving the lorry with
higher LFN level. The results were supported by the objective EEG and
ECG recordings. Tesarz et al. (1997)
investigated 439 persons
working in offices, laboratories and industries. The dominance of LFN at
the workplace was determined by the difference between C- and A-
weighted levels. No person was exposed to noise with C-A differences
greater than 20 dB. Fatigue and tiredness after work increased with
increasing dominance of LFN. Karpova et al. (1970, see above) described
complaints following exposure to LFN: fatigue, feeling of apathy, loss
of concentration, somnolence and depression. Doroshenko (1983, see
above) analysed the anamnestic data and found that the compressor
workers complained about increased irritability, headaches, periodic
vertigo attacks, increased sweating and tiredness, sleep disturbances,
pains in the region of the heart and difficulty in breathing.
Mohr
et al. (1965) carried out systematic investigations with different
types of LFN exposures of very high level. The following subjective
sensations were described:
(1) minor chest wall and body hair vibration due to
a) 124 dB / 10 Hz - 400 Hz broad band noise for 2 minutes (test 1), one of five persons without ear protection
b) 114 dB - 133 dB / 35 Hz - 140 Hz octave band noise for 2 minutes (test 2), one of five persons without ear protection
c) 144 dB / 4 Hz - 4 kHz broadband noise for 1 minute (test 3), no information about wearing of ear protection
(2) "awareness of respiratory action" during test 3
(3)
sensation of moderate chest wall vibration, hypopharyngeal fullness
(gagging), perceptible visual field vibration, prolonged post-exposure
fatigue caused by 143 dB - 145 dB / 10 Hz - 60 Hz narrow band noise
(tests 12, 13, 14), no information about wearing of ear protection
(4)
abdominal wall vibration due to 150 dB - 154 dB / 10 Hz - 20 Hz narrow
band noise for 2 minutes (tests 9, 10 and 11) and nostril vibration
during test 10 (5 Hz - 10 Hz), no information about wearing of ear
protection
The highest pure tone exposures produced with the help
of a siren were used to check the voluntaries tolerance threshold (140
dB - 154 dB / 40 Hz - 100 Hz, 3 subjects, test 16). All three subjects
wore ear protection. It was decided to stop the exposure, because the
following alarming responses occurred: (1) transient headache (one
subject only) at 50 Hz / 153 dB, (2) coughing, substernal pressure,
choking respiration, salivation, pain on swallowing, hypopharyngeal
discomfort, giddiness, testicular aching (one person only) at 153 dB /
60 Hz and 150 dB / 73 Hz, (3) mild nausea, giddiness, subcostal
discomfort, cutaneous flushing, tingling at 153 dB / 100 Hz. All study
participants suffered from evident post-exposure fatigue.
Performance
Evans
et al. (1972, see above) presented a random sequence of illuminated
shapes which had to be recognised by the subjects. Relatively low levels
(115 dB) caused a 30% - 40% increase of the choice reaction time.
Harris et al. (1978, see above) revealed no significant effects on a
serial search task and a complex counting task for the infrasound
exposure in comparison with the 110 dB background noise. Wysocki et al.
(1980, see above) also used a serial search task and analysed the
threshold of speed for the stimuli presentation. A significantly
increased threshold was found in the exposed group. Waye et al. (2002,
see above) obtained a significantly decreased subjectively judged
working capacity due to LFN compared with the reference noise.
Additionally, LFN caused a more pronounced deterioration of highly
demanding task performance (proof-reading task and verbal grammatical
reasoning task). For both tasks, subjects highly sensitive to noise
showed a poorer task performance. This effect could not be found for
routine-type tasks (see also Bengtsson et al. 2000 and Waye et al.
2000). Karpova et al. (1970, see above) mentioned lengthened visual
motor responses to stimuli. Benignus et al. (1975) exposed 27 subjects
to broadband noise (11.5 Hz - 44 Hz and 91 Hz - 350 Hz, both 80 dB,
control condition: no exposure). The rate of misses in a vigilance task
increased significantly during both noise conditions. Schust et al.
(2002) exposed 12 subjects to a LFN spectrum recorded on the bridge of a
ferry boat (main energy in the range of 10 Hz - 100 Hz, 81 dB - 99 dB)
and a reference noise with a flat spectrum (55 dB - 65 dB for all 1/3
octaves up to 20 kHz) recorded in the machine room. Both noise
conditions had the same A-weighted level of 70 dB(A) for 48 minutes. A
barely significant difference was found for the sum score of
"concentration" and "alertness" rated by questionnaire. A greater impact
of LFN was obvious. Moreover, the detection time (signal detection
test) shortened during the reference noise exposure, whereas it remained
constant during the LFN period. This difference approached
significance.
A number of publications deal with the so called
Vibroacoustic Disease (VAD - see Aviation, Space, and Environmental
Medicine 70 (1999) 3, section II, Supplement). In view of this issue,
Gomes et al. (1999) examined 40 workers who have been employed as
aircraft technicians for 22 years in average and 30 educationally and
age-matched controls. The exposure was assumed to be more than 90 dB in
the range below 500 Hz. Significant differences occurred only in one
sub-scale of the two applied performance tests, where the controls had
better results. However, the P300 event-related brain potential showed
significantly prolonged latencies and significantly smaller amplitudes
in the occupationally exposed group.
Discussion | |  |
Hypothetically,
effects of LFN may be mediated trough different ways: (1) vibration of
the eardrum, leading to a hearing sensation through pressure changes in
the cochlear endolymph, activation of the hair cells and the acoustic
nerve, (2) direct energetic transmission of the airborne acoustical
sound waves into the cochlear endolymph or into the acoustic nerve, that
means pressure changes in the cochlear endolymph without involvement of
the eardrum or excitation of the acoustic nerve without involvement of
the eardrum and the endolymph, but both leading to a hearing sensation
(a rather unlikely hypothesis as long as the middle ear is intact
because of the higher impedance of this way in comparison with (1); more
probable, (2) might act additionally to (1)), (3) excitation of the
vestibular system, (4) excitation of receptors and/or nerve fibres in
the skin or in any other kind of tissue or blood vessels within the
organism - e.g. the optic nerve, mechanoreceptors and baroreceptors
responsible for the control of the blood pressure - by direct energetic
transmission of the airborne acoustical sound waves. Following these
hypotheses, on one side, a determination of the thresholds of hearing,
of vibrotactile perception and of aural pain [Figure - 1]
requires a conscious sensation of the stimulus. On the other hand,
other biological reactions could arise even below the perception
thresholds. Particularly, the pathways (3) and (4) do not presuppose
conscious sensations. Bearing these pathways in mind, the scientific
results shall be discussed now.
Aural effects
The
function of the hearing organ was checked with the tone audiometry in
the presented studies. This method is a subjective one. It requires an
active involvement of the study participant and a conscious hearing of
LFN. Acoustic stimuli affecting the hearing organ are supposed to
activate the ways (1) or (2) mentioned above. That means, they
accordingly cause a hearing sensation. The methods for the estimation of
the resting hearing threshold and for the measurement of temporary or
permanent shifts of the hearing threshold (TTS or PTS) activate the same
sensory pathways. Consequently, it may be assumed that the TTS or PTS
estimated by audiometry appear due to acoustic stimuli above the
individual hearing threshold. The results unquestionably confirm this
assumption. In [Figure - 2],
the only exposure condition below the hearing threshold which was
associated with a threshold shift was part of a LFN spectrum (Doroshenko
et al. 1983). Perhaps, the acoustic energy above the hearing threshold
was responsible for the effect. The combined steady noise within the
hearing frequency range could also explain the obtained threshold shift.
Apart
from that, there is a need for further scientific research. No
publication described LFN-induced threshold shifts in the relevant low
frequency hearing range. The measured audiometry frequencies were not
reported in most of the papers. The resting hearing thresholds of the
subjects were not given for the low frequencies. It can be assumed,
therefore, that only the conventional audiometry range from about 500 Hz
to 6 kHz was checked.
Additionally, the use of objective methods
like the recording of brainstem potentials or otoacoustic emissions
could lead to different results concerning the hearing threshold or the
impairment of hearing due to LFN exposure.
A sensation of
"pressure in the ear" might be caused by baroreceptors located in the
tissue of the ear channel or in the adjacent tissue around the cortical
organ (pathway (4)). From this point of view, the sensation could occur
below the hearing threshold. On the other hand, as mentioned above, this
pathway is quite implausible as long as the middle ear works properly.
The results support these doubts. Only Mohr et al. (1965) noticed a
sensation of pressure in the ear due to 129 dB / 1 Hz as a part of a LFN
spectrum (see [Figure - 2]).
Perhaps, the acoustic energy of the sound above the hearing threshold
caused this perception. These results correspond with the findings of
Landstroem et al. (1983). Two of the 10 deaf subjects could "feel the
sound through their ears", based on a sensation of vibrations and
pressure changes in the ear ("pseudoauditory air conduction"), but only
when the levels exceeded 120 dB (4 Hz - 25 Hz). These sound pressure
levels lie above the hearing threshold.
The studies of Johnson
(1973, cited in Johnson 1982) with very high sound levels remain to be
discussed. There were two subjects exposed above the threshold of aural
pain without reporting on TTS or pain. But the original paper did not
talk about these experiments, so that the results cannot be judged.
Maybe, the subjects wore ear protection. Johnson (1982) described an
experiment he experienced himself. He listened to 172 dB / 4 Hz for less
than 30 seconds by using an earcup. He felt no pain but a "massaging"
of the tympanic membrane. He concluded, that damage could occur to the
middle ear without previous pain. Summarising the present knowledge,
this conclusion could be supported.
Non-aural effects
Subjective complaints
A
conscious perception is to be presupposed for the sensation of
vibration of different parts of the body. Therefore, such effects should
arise around or above the threshold of vibrotactile perception [Figure - 1]. The present results confirm this assumption (see [Figure - 3],[Figure - 4]).
However, sensations concerning the vestibular system (pathway (3)) or
other sensors including vibration sensors (pathway (4)) might appear
below the hearing threshold. Actually, some investigations revealed
complaints like somnolence, irritability, tiredness, tense and
restlessness, below or at least near the hearing threshold (Ising et al.
1979 and 1980, Karpova et al. 1970, see [Figure - 3],[Figure - 4]). The other sound levels, which were associated with subjective complaints and ranged below the hearing threshold in [Figure - 3],[Figure - 4]
(Doroshenko et al. 1983, Landstroem et al. 1988), were part of a
broader LFN-spectrum. Nevertheless, the revealed complaints - like
increased fatigue, irritability, headaches, periodic vertigo attacks,
increased sweating and tiredness, sleep disturbances, pains in the
region of the heart and difficulty in breathing - might be caused by the
lower frequency parts of the spectrum, too.
Vascular, respiratory and endocrine effects, balance and visual disturbance
As
already argued above, these effects might emerge due to exposure below
the hearing threshold, because the pathways (3) and (4) perhaps do not
presuppose a conscious perception of the LFN by the cortical organ. The
findings seem to support this deduction. Danielson et al. (1985) and
Karpova et al. (1970) reported on changes in blood pressure, respiration
rate, EEG patterns and heart rate caused by exposure below or near the
hearing threshold (see [Figure - 3]).
The low level exposures used by Wysocki et al. (1980), Waye et al.
(2002) and Doroshenko et al. (1983) also comprised acoustic energy above
the hearing threshold. Nevertheless, the reported effects - like
changes in heart rate, peripheral vascular blood flow, skin temperature,
cortisol levels and vestibular functions - could have been triggered by
the lower parts of the frequency spectrum.
Performance
The
same arguments as mentioned above are assumed to be valid for the
influence of LFN on the performance. Evans et al. (1972) and Karpova et
al. (1970) found lengthened visual motor responses caused by LFN
exposures below or near the hearing threshold. The impairment of
performance obtained by Wysocki et al. (1980), Waye et al. (2002) and
Benignus et al. (1975) could be generated by the lower frequency parts
of the spectrum below the hearing threshold, too (see [Figure - 3]).
Conclusions | |  |
There
is a clear frequency dependence of the hearing threshold in the low
frequency range with a steep slope compared with the middle frequency
range. On the other hand, the threshold of aural pain is less dependent
on the frequency. It varies from 130 dB at middle frequencies around 1
kHz to 160 dB at very low frequencies. Consequently, the dynamic range
between the thresholds of hearing and of sensation of pain diminishes
with decreasing frequency [Figure - 1].
Moreover, the equal loudness contours narrow with diminishing frequency
(Robinson et al. 1956). Because of the reduced dynamic range, small
changes of the LFN stimulus can cause great differences of the response
effects. Therefore, different individual effects may be expected even
under similar exposure conditions. This should be taken into account by
the setting of limits concerning the health risks. Sufficient safety
margins are recommended. The use of a frequency weighting with an
attenuation of the low frequencies (e.g. G-weighting) does not seem to
be appropriate for the evaluation of the health risks caused by LFN up
to 100 Hz. There is no scientific evidence for an association between a
frequency-weighted sound pressure level and the biological effect. It
may be proposed to measure third octave band spectra or narrow band
spectra. A comparison with the known human responses caused by the
measured levels and frequencies could help to evaluate the health risks.
The
knowledge reviewed in this paper is mainly based on laboratory studies.
Only a few field studies were found. A comprehensively critical view of
methodological issues concerning these types of investigations was
given by Berglund et al. (1996). Moreover, the authors provided
recommendations for further studies. The proposals are still up to date.
They shall to be only shortly noticed here:
(1) investigation of effects of different frequency spectra
(2) prolonged exposures in laboratory studies
(3) realisation of longitudinal epidemiological studies
(4) use of the rare occasions of noise source changes for epidemiological studies (e.g. opening of new freeways or airports)
(5) measurement and statistical control of confounding factors
(6) consideration of individual differences (7) taking into account various risk groups
(8) development of standardised techniques to measure LFN
(9) realisation of laboratory studies of various features of noise signals
(10) investigation of the relative contributions of LFN, impulsiveness and tonality to the human response
(11) study of the relative importance of vibration and rattle versus LFN
(12) development of methods for LFN attenuation and control measurement technology
Additionally, some more proposals could be made as a result of this review:
(1)
The measurement of the individual hearing threshold and the
vibrotactile threshold for airborne LFN in the exposed frequency range
could be helpful for the judgement of the obtained effects. The
objective methods like the recording of brainstem potentials or
otoacoustic emissions should to be considered, too.
(2) In
laboratory experiments, an exposure via loudspeakers (whole body
exposure) probably better reflects the practically environmental
conditions than the use of headphones alone. On the other hand,
headphones can be employed to distinguish the ways of sensation of LFN.
The exposure to LFN near or above the vibrotactile threshold via
loudspeaker could lead to different effects compared with pure headphone
exposure.
(3) Experiments with hearing versus deaf persons or
with/without ear protection ought to be carried out to clarify the
pathways of LFN sensation.
(4) The hearing threshold shifts in the frequency range of the exposure should be determined (see discussion of aural effects).
(5)
The level of the airborne noise and the body vibration caused by this
airborne noise or by other sources ought to be measured simultaneously.
Appropriate methods for laboratory and field studies were described by
Takahshi et al. (1999 and 2001) and Smith (2002).
(6) Combined
effects of LFN and body vibration should be taken into account. There is
some evidence of mutual effects of both factors at least concerning the
thresholds of hearing and vibrotactile sensation (Sueki et al. 1998)
and the influence of vibration on the equal loudness level contours
(Bellmann et al. 1999).
(7) A cause-effect modelling ought to be
tried to analyse the transmission of the acoustic energy from the input
into the body to the structures containing sensors, like the Membrana
basilaris, the adjacent tissue around the Nervus cochlearis, the
vestibular system, the skin layers, the inner organs, muscles etc.
Bearing this in mind, individual features could be of interest, e.g.
anthropometric characteristics like height, subcutaneous fat layer,
muscularity etc.. For example, Takahshi et al. (1999) found a negative
correlation between the measured noise-induced vibration and the
subject´s body mass index.
(8) Different risk groups like children or pregnant women should be considered (for arguments see point 7)[47].
References | |  |
1. | Alford,
B. R.; Jerger, J. F.; Coats, A. C.; Billingham, J.; French, B. O.;
McBrayer, R. O. (1966) Human tolerance to low frequency sound.
Transactions - American Academy of Ophthalmology and Otolaryngology 70 (1): 40 - 47 |
2. | Bellmann, M. A.; Reckhardt, C.; Mellert, V. (1999) Sound and vibration at low frequencies. Acta Acustica 85, Suppl. 1(Joint meeting: ASA/EAA/DEGA): 371 |
3. | Benignus, V. A.; Otto, D. A. (1975) Effect of lowfrequency random noises on performance of a numeric monitoring task. Perceptual and motor skills 40 (1): 231 - 239 |
4. | Bentsson, J.; Waye, K. P.; Kjellberg, A.; Benton, S. (2000) Low frequency noise "pollution" interferes with performance. In Proceedings of the Internoise 2000,
29th International Congress on Noise Control Engineering, Cassereau,
D., eds. Societe Francaise d'Acoustique, Nice, pp 2859 -
2862 |
5. | Berglund, B.; Hassmen, P. (1996) Sources and effects of low frequency noise. J. Acoust. Soc. Am. 99 (5): 2985 - 3002 |
6. | Broner, N. (1978) The effects of low frequency noise on people - a review. J. Sound Fib. 58 (4): 483 - 500 |
7. | Corso, J. F. (1958) Absolute thresholds for tones of low frequency. Am. J. Psychol. 71: 367 - 374 |
8. | Danielsson, A.; Landstroem, U. (1985) Blood pressure changes in man during infrasonic exposure. Acta Medica Scandinavica 217 (5): 531 - 535 |
9. | Doroshenko,
P. N.; Stepchuk, I. D. (1983) Health related assessment of combined
effect of infrasound and lowfrequency noise on the acoustic and
vestibular analyser of compressor operators. Gigiena Truda i Professional'nye Zabolevaniya 1: 35 - 38, English translation in Noise and Vibration Bulletin (Nov. 1983): 192 - 194 |
10. | Evans, M. J.; Tempest, W. (1972) Some effects of infrasonic noise in transportation. J. Sound Fib. 22 (1): 19 - 24 |
11. | Gierke, von, H. E.; Nixon, C. W. (1976) Effects of intense Infrasound on Man. In Infrasound and Low frequency vibration. Tempest, W., eds. Academic London, pp 115 - 150 |
12. | Gomes
L. M.; Martinho Pimenta A. J.; Castelo Branco N. A. (1999) Effects of
occupational exposure to low frequency noise on cognition. Aviation, Space, and Environmental Medicine 70 (3, Section II): A115 - A118 |
13. | Harris, C. S.; Johnson, D. L. (1978) Effects of infrasound on cognitive performance. Aviation, Space, and Environmental Medicine 49 (4): 582 - 586 |
14. | Harris, C. S.; Sommer, H. C.; Johnson, D. L. (1976) Review of the effects of infrasound on man. Aviation, Space, and Environmental Medicine 47: 430 - 434 |
15. | Ising,
H. ; Wittke, C. (1979) Auswirkungen mehrstundiger Infraschallexposition
auf Versuchspersonen (Reactions of test persons long-term exposed to
infrasound). Forum Stddte Hygiene 30: 49 - 52 |
16. | Ising, H. (1980) Psychological, ergonomical and physiological effects of long-term exposure to infrasound and audiosound. Noise and Vibration Bulletin (Aug. 1980): 168 -174 |
17. | Jerger, J.; Alford, B.; Coats, A.; French, B. (1966) Effects of very low frequency tones on auditory thresholds. J. Speech Hear. Res. 9: 150 - 160 |
18. | Johnson, D. L. (1982) Hearing hazards associated with infrasound. In New perspectives on noise-induced hearing loss. Hamernik, R. P.; Henderson, D.; Salvi, R., eds. Raven Press, New York, pp 407 - 421 |
19. | Johnson, D. L. (1980) The effects of high level infrasound. In Proceedings of the conference on low frequency noise and hearing. Moller, H.; Rubak, P., eds. Aalborg University Center, Aalborg, pp 47 - 60 |
20. | Johnson, D. L. (1973) Various aspects of infrasound. In Proceedings of Colloquium of Infrasound. Pimonow, L., eds. Centre National de la Recherche Scientifique, Paris, pp 337 -356 |
21. | Karpova
N. I.; Alekseev S. V.; Erokhin V. N.; Kadyskina E. N.; Reutov O. V.
(1970) Early response of the organism to low-frequency acoustical
oscillations. Noise and Vibration Bulletin 11 (65): 100-103 |
22. | Landstroem, U.; Haggqvist, S. L.; Lofstedt, P. (1988) Low frequency noise in lorries and correlated effects on drivers. Journal of Low Frequency Noise and Vibration 7 (3): 104109 |
23. | Landstroem, U.; Lundstroem, R.; Bystroem, M. (1983) Exposure to infrasound - perception and changes in wakefulness. J. of Low Frequency Noise and Vibration 2: 1 - 11 |
24. | Landstroem, U.; Pelmear, P. L. (1993) Infrasound - A short review. J. of Low Frequency Noise and Vibration 12 (3): 72 - 74 |
25. | Mills,
J. H.; Osguthorpe, J. D.; Burdick, C. K.; Patterson, J. H.; Mozo, B.
(1983) Temporary threshold shifts produced by exposure to low-frequency
noises. J. Acoust. Soc. Am. 73 (3): 918 - 923 |
26. | Mohr, G. C.; Cole, J. N., Guild, E.; Gierke, von, H. E. (1965) Effects of low frequency and infrasonic noises on man. Aerospace Med. 36: 817 - 827 |
27. | Nixon, C. W. (1973) Human auditory response to intense infrasound. In Proceedings of the Colloquium of infrasound. Pimonow, L., eds. Centre National de la Recherche Scientifique, Paris, pp 315 - 336 |
28. | Robinson, D. W.; Dadson, R. S. (1956) A re-determination of the equal-loudness relations for pure tones. British J. Appl. Physics 7: 166 - 181 |
29. | Sataloff, R. T.; Sataloff, J. (1993) Occupational hearing loss. New York Marcel Dekker |
30. | Schust,
M.; Eggert, U. (2002) Wirkung von arbeitsplatztypischen tieffrequenten
Gerauschen (Effects of low frequency noise typical for workplaces). In
Tagungsband der 28. Jahrestagung ffur Akustik der Deutschen
Gesellschaft ffur Akustik (Proceedings of the 28th Annual Acoustical
Congress of the German Society of Acoustics), eds. DEGA e.V., Bochum, pp 340-341 |
31. | Slarve R. N.; Johnson D. L. (1975) Human whole - body exposure to infrasound. Aviation, Space, and Environmental Medicine 46 (4): 428 - 431 |
32. | Smith, S. D. (2002) Characterizing the effects of airborne vibration on human body vibration response. Aviation, Space, and Environmental Medicine 73 (1): 36 - 45 |
33. | Sueki,
M.; Noba, M.; Nakagomi, M.; Kubota, S.; Okamura, A.; Kosaka, T.;
Watanabe, T.; Yamada, S. (1989) Study on Mutual Effects of Low Frequency
Noise and Vibration. Journal of Low Frequency Noise and Vibration 8: 66 - 75 |
34. | Takahashi, Y.; Yonekawa, Y.; Kanada, K.; Maeda, S. (1999) A pilot study on the human body vibration induced by low frequency noise. Industrial Health 37: 28 - 35 |
35. | Takahashi, Y.; Yonekawa, Y.; Kanada, K. (2001) A new approach to assess low frequency noise in the working environment. Industrial Health 39: 281 - 286 |
36. | Takigawa, H.; Hayashi, F.; Sugiura, S.; Sakamoto, H (1988) Effects of infrasound on human body sway. Journal of Low Frequency Noise and Vibration 7 (2): 66 - 73 |
37. | Tempest, W. (1976) Infrasound and Low frequency vibration. Academic London |
38. | Tesarz, M.; Kjellberg, A.; Landstroem, U.; Holmberg, K. (1997) Subjective response patterns related to low frequency noise. Journal of Low Frequency Noise and Vibration 16 (2): 145 - 149 |
39. | Tonndorf, J. (1950) The influence of service on submarines on the auditory organ. Appendix to German aviation medicine in World War II. Chapter DH. eds. Department of the Airforce, Washington D.C. |
40. | Verzini,
A. M.; Ortiz Skarp, A. H.; Nitardi, H.; Fuchs, G. L., (1999) A
laboratory experiment on very low frequency sound effects. Appl. acoustics 57: 69 - 77 |
41. | Waye,
K. P.; Rylander, R.; Bengtsson, J.; Hucklebridge, F.; Evans, P.; Clow,
A. (2000) Does low frequency noise during work induce stress?. In Proceedings of the Internoise 2000,
29th International Congress on Noise Control Engineering, Cassereau,
D., eds. Societe Francaise d'Acoustique, Nice, pp 2871 -
2874 |
42. | Waye,
K. P.; Bengtsson, J.; Rylander, R.; Hucklebridge, F.; Evans, P.; Clow,
A. (2002) Low frequency noise enhances cortisol among noise sensitive
subjects during work performance. Life sciences 70 (7): 745 - 758 |
43. | Westin, J. B. (1975) Infrasound: A short review of effects on man. Aviation, Space, and Environmental Medicine 46: 1135 - 1140 |
44. | Whittle, L. S.; Collins, S. J.; Robinson, D. W. (1972) The audibility of low frequency sounds. J. Sound Fib. 21: 431 - 448 |
45. | Wysocki,
K. ; Schultz, K. ; Wieg, P. (1980) Experimentelle Untersuchungen zum
Einflul3 von Infraschalldruck auf den Menschen (Experimental studies of
the influence of infrasonic noise on the human organism). Z. f. die ges. Hyg. und ihre Grenzgebiete 26 (6): 436 - 440 |
46. | Yeowart, N. S.; Bryan, M. E.; Tempest, W. (1967) The monaural MAP threshold of hearing at frequencies from 1.5 to 100 c/s. J. Sound Fib. 6: 335 - 342 |
47. | Yeowart, N. S.; Evans, M. J. (1974) Thresholds of audibility for very low frequency pure tones. J. Acoust. Soc. Am. 55 (3): 814 - 818 |

Correspondence Address: M Schust Federal Institute for Occupational Safety and Health, Nöldnerstr. 40 – 42, D – 10317 Berlin Germany
 Source of Support: None, Conflict of Interest: None  | Check |
PMID: 15273025 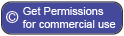 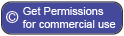
[Figure - 1], [Figure - 2], [Figure - 3], [Figure - 4] |
|
This article has been cited by | 1 |
A software library for active control of automotive engine noise |
|
| Every, M.R. and Macdonald, K. | | SAE Technical Papers. 2013; 4(040110) | | [Pubmed] | | 2 |
Assessment of noise induced hearing loss of the mine workers of a chromite mines at Sukinda, Orissa, India |
|
| Kerketta, S. and Gartia, R. and Bagh, S. | | Noise and Vibration Worldwide. 2012; 43(2): 28-36 | | [Pubmed] | | 3 |
Occupational hearing loss of the workmen of an open cast chromite mines |
|
| Kerketta, S. and Gartia, R. and Bagh, S. | | Indian Journal of Occupational and Environmental Medicine. 2012; 16(1): 18-21 | | [Pubmed] | | 4 |
Exposure to industrial wideband noise increases connective tissue in the rat liver |
|
| Oliveira, M.J.R. and Freitas, D. and Carvalho, A.P.O. and Guimarães, L. and Pinto, A. and Ãguas, A.P. | | Noise and Health. 2012; 14(60): 227-229 | | [Pubmed] | | 5 |
Chronic exposure to low frequency noise at moderate levels causes impaired balance in mice |
|
| Tamura,
H. and Ohgami, N. and Yajima, I. and Iida, M. and Ohgami, K. and Fujii,
N. and Itabe, H. and Kusudo, T. and Yamashita, H. and Kato, M. | | PLoS ONE. 2012; 7(6) | | [Pubmed] | | 6 |
The possible influence of noise frequency components on the health of exposed industrial workers - A review |
|
| Veerappa, M.P.K., Venugopalachar, S. | | Noise and Health. 2011; 13(50): 16-25 | | [Pubmed] | | 7 |
Auditory cortex stimulation by low-frequency tones-An fMRI study |
|
| Dommes, E., Bauknecht, H.C., Scholz, G., Rothemund, Y., Hensel, J., Klingebiel, R. | | Brain Research. 2009; 1304: 129-137 | | [Pubmed] | | 8 |
Non-auditory health effects among air force crew chiefs exposed to high level sound |
|
| Jensen, A., Lund, S., Lücke, T., Clausen, O., Svendsen, J. | | Noise and Health. 2009; 11(44): 176-181 | | [Pubmed] | | 9 |
Intrasound
and low-frequency noise - A theme for environmental-related health
protection in Germany? Communication of the commission "Methods and
quality assurance in environmental medicine" [Infraschall und
tieffrequenter Schall - Ein Thema für den umweltbezogenen
Gesundheitsschutz in Deutschland? : MMitteilung der Kommission "Methoden
und Qualiẗatssicherung in der Umweltmedizin"] |
|
| | | Bundesgesundheitsblatt - Gesundheitsforschung - Gesundheitsschutz. 2007; 50(12): 1582-1589 | | [Pubmed] | |
|
|
 |
 |
|
|
|